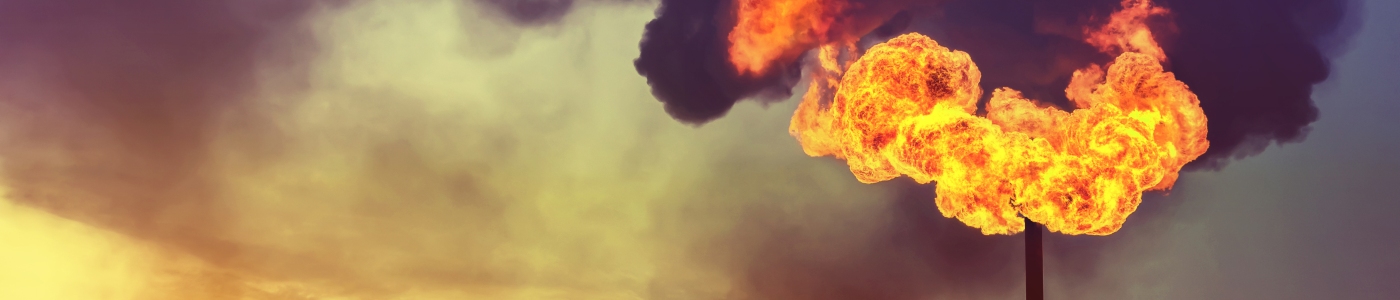
Reducing methane emissions in the atmosphere is the fastest way to slow climate change
Sensing and Imaging for Climate Change
To tackle Climate Change, it is necessary for both industry and government organisations to have accurate, widespread access to monitoring solutions that can show the emissions, condition, and sustainability of our society.
QuantIC has developed a range of imaging solutions that address major areas of climate impact. These include:
- monitoring gas emissions, such as methane and hydrogen
- structural health monitoring within challenging environments such as underwater
- enabling better product longevity for renewable energy sources
Find out more about QuantIC's research and the commercialisation of our technology in this field.
Quantum Imaging for Climate Change
Discover how researchers at the University of Bristol have developed highly sensitive gas imaging technology
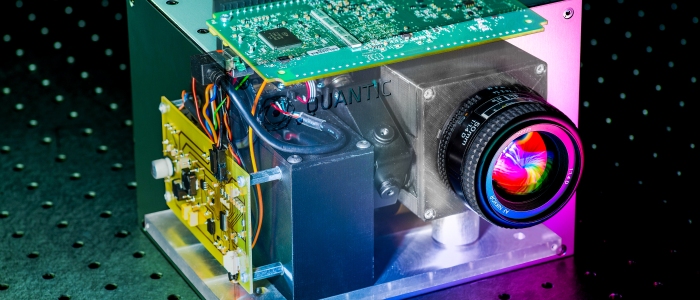
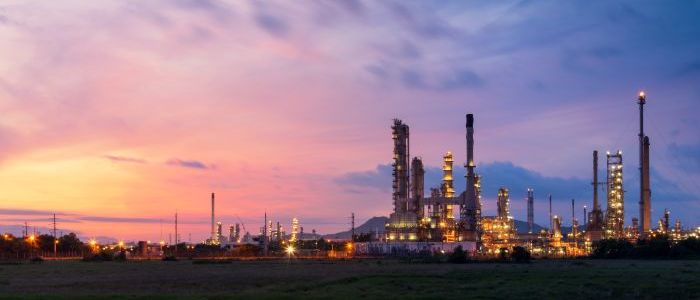
QuantIC technology for climate change
IMAGING WITH UNDETECTED PHOTONS
Central to all conventional imaging is the notion that the light which illuminates the object is the light that is detected by the image sensor.
In quantum science, this light can be linked (entangled) to light of a different wavelength. Consequently, the image encoded on infra-red light used to illuminate an object can, after transmission through the object, be transferred to visible light. The object is always illuminated by the infra-red light but the image can be observed in the visible signal.
QuantIC contributions to the field include using new structured materials to generate entangled photons further into the infrared and targeting the wavelengths used to detect hydrocarbon gases.
If such systems are realised then it becomes possible, using standard visible (i.e. silicon-based) cameras, to image at many wavelengths across the electromagnetic spectrum, potentially reaching as far as the terahertz regime for application in clinical and pharmaceutical science.
GAS SENSING WITHOUT DETECTION
Gases often absorb in both the short-wave (1-2μm) mid-wave (3-5μm) infrared regions of the spectrum. The absorption in the mid-wave is typically much higher than that in the short-wave meaning that, in principle, lower concentrations of gas can be detected when operating in the mid-wave infrared. However, the corresponding mid-wave detectors are much worse than their short-wave counterparts meaning that the overall system performance is compromised.
QuantIC researchers are using an entangled photon-pair source that produces photon-pairs at two different wavelengths and, thanks to quantum interference, we plan to measure the stronger mid-wave absorption using the sensitive short-wave detector. If successful, this work could transform the detection of hydrocarbon and similar gases. Making possible the sensing of lower concentrations of gas by up to two-orders of magnitude.
NANOPHOTONIC SOURCES
Key to many quantum systems are sources of light that produce entangle pairs of photons. These photons are created using non-linear crystals which convert photons from a laser into photon pairs of longer wavelengths. The range of wavelengths possible are restricted by the properties of crystals that can be grown.
At QuantIC we are using arrays of nanostructures that can be designed to create a broader range of wavelengths to produce entangled photons at precise wavelengths in the infra-red, or longer, for specific sensing requirements such as the detection of greenhouse gases.
Beyond being a pivot component in quantum optics, even as a classical source, this approach might yield sources of light of unparalleled brightness for gas sensing applications.
INSB APD DETECTOR ARRAYS
For most detector types, the range of wavelengths over which they are sensitive depends on the semiconductor material from which they are made.
We are developing 64x64 element detector arrays based on indium antimonide (InSb) for imaging application in the mid-infra-red. By using these devices in avalanche mode we can reach extreme sensitivity, approaching that required to measure single photons.
This wavelength range is not only ideal for the detection of hydrocarbons and other gases but can be used for material recognition in recycling and other industrial screening applications.
WAVELENGTH TRANSFORMATION CAMERA
The goal is to bring visible detector performance to infrared imaging systems.
We use non-linear optical materials to convert infrared light into near-visible light so that it can be imaged using conventional, cheap, high-performance cameras based upon silicon technology. To date, no one has managed to do this with both high efficiency and in a multi-pixel configuration.
QuantIC contributions to the field include, putting the non-linear material in a waveguide configuration to increase the conversion efficiency and using multiple waveguides and scanning systems in various configurations to make a multi-pixel device. The waveguide configuration can reach a conversion efficiency for infrared to visible light of 40%.
This technology will have use in domestic and retail sites for Energy Performance Certificate surveys.
SINGLE-FIBRE ENDOSCOPE
Traditional endoscopes are based on bundles of many thousands of optical fibres, one fibre for every pixel in the image.
A less intrusive endoscope could be based on a single-fibre the width of a human hair. However, when an image is transmitted along a single-fibre, cross-talk between modes, scrambles the light to make the image unrecognisable.
At QuantIC we are making single fibre endoscopes possible. Using advanced computing, beam shaping, and photon timing allows a 3D image to be extracted from this noise. Such systems could be used in EPC surveys, and manufacturing inspections to promote ‘right first time’, minimising waste and improve product longevity.
UNDERWATER IMAGING
Imaging through clear and clean water can be quite straightforward. However, once the water becomes turbid, the high degree of back-scattered light saturates the detector and the image can no longer be recognised. One approach to overcoming this backscatter is to use short-pulse illumination and time-resolved single-photon detection so that the returned photons can be seen above the back-scattered background level.
We are using both SPAD detector arrays and single-detector, raster scanned systems to suppress backscatter from the recorded images. In both cases the timing resolution corresponds to a few millimetres in depth and by rejecting all the other photons we have shown imaging at over 9 scattering lengths (measured one way) at ranges of several metres.
Such systems have significant application within sub-sea operations, for example in offshore installation inspection and terrain mapping and hence navigation. We are presently building a demonstration unit for trials in real-world conditions.