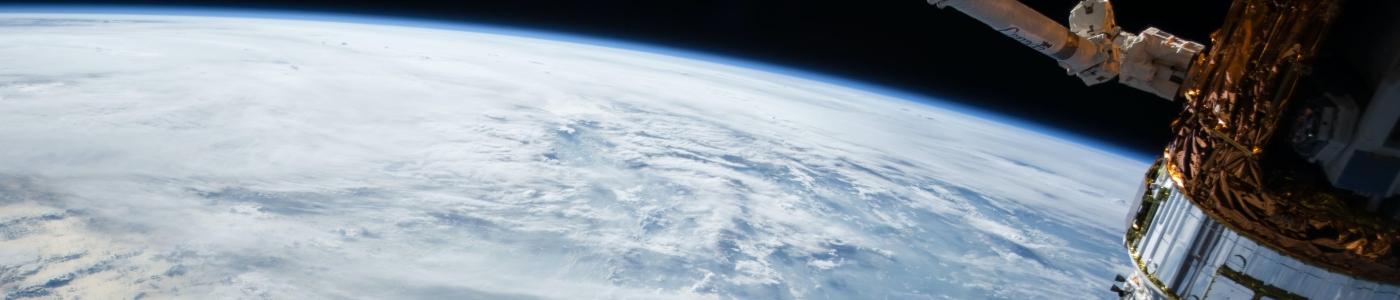
Satellites and low orbit technology are vital for earth observation, communications, and tracking
Sensing and Imaging for Space
Satellites and low orbit technology are vital for a wide range of terrestrial applications, from communications and weather systems tracking to navigation and earth observation.
QuantIC researchers are developing superconducting single-photon detectors. These devices set the gold standard for low noise, high speed, high-efficiency, single photon detection in a broad spectrum of quantum technology applications. Key application areas include single photon LIDAR and ground-to-space sensing applications.
Clusters of nanosatellites can communicate in space using LED-based transceivers developed by QuantIC. They benefit from ultra-low power consumption, small footprint and high resilience to background noise. Such constellations require self-alignment and communication systems without greatly increasing the power consumption of the satellite unit.
QuantIC technology for Space
SUPERCONDUCTING DETECTORS
In pushing the limits of single-photon detection, superconducting detectors offer an important alternative to semiconductor photon counters. A superconducting nanowire, cooled to a few degrees Kelvin, acts as a high-speed switch in response to infrared photons. The absorbed photon perturbs current flow in the superconducting nanowire and can be detected as a fast voltage pulse. Photon detectors based on superconducting nanowires are sensitive to the widest possible range of wavelengths, can reach the ultimate sensitivity (≈90%) with negligible dark counts and reach the fastest response times (≈10ps).
QuantIC researchers are making these photon detectors more accessible by developing compact fridges so that they can be widely deployed in imaging trials with QuantIC partners. This work is being undertaken in conjunction with the Quantum Communications Hub. Detectors of this kind can be used in the monitoring of Photodynamic therapy.
REAL TIME 3D IMAGING
Most conventional cameras only see in 2D. By using real-time technology, we can time how long it takes light to bounce back from the object, producing a 3D image. This is prime technology for depth imaging through complex and challenging conditions such as providing visibility in low atmospheric situations.
Building upon the work in QuantIC phase one, we are using pulsed illumination and SPAD sensor arrays to record the range of each image pixel (>10,000 pixels) simultaneously. Results have shown centimetre depth resolution at 10s or even 100s metre range and frame rates up to 30Hz. The sensor data is denoised using sophisticated data processing to give 3D representations of the surrounding environment.
QUANTUM RADAR/LIDAR
Lidar and radar use the time of flight of light or radio-wave pulses respectively to measure the distance to the backscattering object. However, in many cases the regular nature of these pulses means that such systems can be confused either by accident or by deliberate intervention.
At QuantIC we are using entangled photons to create two identical, yet within themselves random, light beams. One of these beams illuminates the target and the other acts a unique and unpredictable reference against which to validate any return signal. Such systems eliminate range ambiguity and cannot be spoofed by someone seeking to mislead the system. Our latest work relies on randomness in both time and wavelength meaning that the illumination beam can be weaker than the background light making the approach covert.
Such systems could be relevant within space and low orbit, providing the ability to distinguish objects quickly e.g. satellites or space debris.
SPATIO-TEMPORALLY CORRELATED MULTI-MODE OPERATION
Typically, imaging systems make use of ambient light or uniform illumination of a scene by laser or other artificial light sources, recovering spatial information with pixelated detector arrays. Alternatively, patterns can be projected onto the scene and the overlap of each pattern with the scene measured. In QuantIC, we use CMOS-interfaced micro-pixel LED arrays with MHz switching speeds for 3D imaging, ranging, and positioning systems, combined with data communications.
This technology is now being pushed into the deep ultra-violet, with interesting prospects for non-line-of-sight scenarios. We are developing new CMOS-controlled LEDs with finer spatiotemporal resolution and continue to improve our 3D imaging methods through optimised processing and fused imaging modes.
CMOS SPAD ARRAYS
Traditional cameras include a sensor chip comprising an array of pixels, each of which measures the number of photons that strike the sensor at that particular position. Instead a SPAD measures not the number of photons but rather the time at which the first photon arrives. If the object is illuminated with a pulse of light then the arrival time of the first photon corresponds to the distance to that pixel – giving a 3D image.
Building upon the work in QuantIC phase one, contributions that we are making to the field include increasing the detection efficiency from <10% to ≈ 60%, improving the timing resolution (≈30ps -> depth resolution ≈5mm) and increasing the number of pixels in the image (256x256).
These cameras can measure the arrival time of every photon, allowing it to build up a 3D image of a scene enhancing optical performance in satellite communication.
CASE STUDY: CLYDE SPACE AND SATELLITE COMMUNICATION
Clusters of nanosatellites can communicate in space using LED-based transceivers developed by QuantIC. They benefit from ultra-low power consumption, small footprint and high resilience to background noise.
QuantIC’s researchers worked with Clyde Space, world leading innovator and supplier of CubeSats and small satellite systems, and the Scottish Centre of Excellence in Satellite Applications to develop a low light level communications and positioning system that operates at the few-photon level, close to the quantum limit.
This technology will allow constellations of satellites to identify their own position relative to other units and to communicate with each other, all at ultra-low power levels, and with form factors compatible with current CubeSat standards.QuantIC’s LED emitter technology and single photon receivers crucially enable this performance.
It also offers a high level of resilience against both DC and AC background noise. Transmitter and receiver are both conveniently interfaced with CMOS digital electronics.
“As the market continues to rapidly develop, there are ever increasing applications of nanosatellite constellations – systems of nanosatellites working together to solve a particular problem. QuantIC’s novel optical communications technology coupled with Clyde Space’s extensive experience in spacecraft design ensures that key commercial constellations missions can be met with system-optimised solutions which are not only responsive to market demand but pioneer industry innovations.” – Andrew Strain, Chief Engineer, Clyde Space.